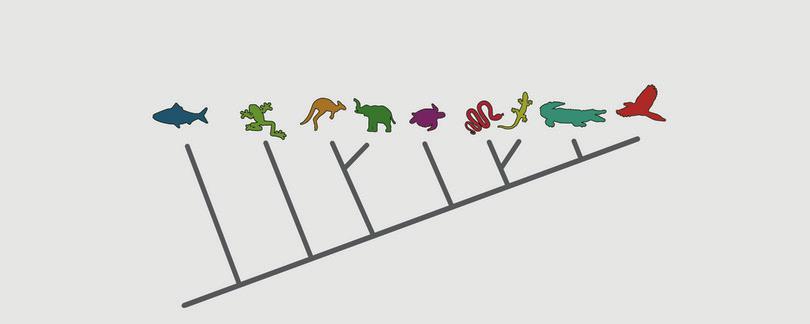
IV Evolution
IV.1 Introduction
In iThink Biology you will come across several questions about the diverse life forms on Earth: why some groups are more diverse than others (Western Ghats and Waterscapes); how one species differs from another (Rice and Rotavirus); how certain organisms have evolved specific adaptations with their host (Figs) and to environmental change (Malaria); or how humans have modified certain species for their own needs (Cotton). The underlying principle for all these patterns and processes is evolution. As the famous Ukranian–American geneticist Theodore Dobzhansky once said, ‘nothing in biology makes sense except in the light of evolution’.
Although we have mentioned terms such as evolution, natural selection and adaptation, we have not addressed them in detail in the chapters. We have written this primer as a set of questions and answers; by doing so, we hope to highlight some basic principles of evolution, as well as address some common misconceptions. This is in no way an exhaustive list of everything that you might want to know about evolution, but it will help you understand the basics.
IV.2 What is evolution?
Charles Darwin made three broad observations about nature: the unity of life, the diversity of life, and the match between organisms and the environment. He said that the organisms that we see today – from bacteria to blue whales, from hummingbirds to humans – are all related to each other. Darwin based this unity of life on his observation of organisms that shared similar characteristics, stating that they might share a common ancestor. We can explain the diversity of life by gradual accumulation of modifications to suit specific environments.1 Evolution is descent with modification.
Life on Earth is approximately 3.5 billion years old. Through descent with modification, the common ancestor has given rise to the breathtaking diversity that we see on Earth today. Life on Earth is grouped into three domains: Bacteria, Archaea and Eukaryota (Figure IV.1). Click on each of the three slides in Figure IV.1 to explore the diversity of life on earth, and how various life forms are related to each other. Find out where humans are placed on the tree of life. We will look into phylogenetic trees in more detail later in this primer.
Charles Darwin was not the first scientist to suggest that life has evolved. Fossils showed that many forms of life had existed in the past and are now extinct. However, many fossils share similarities with species that exist at present. Many scientists, including Darwin’s grandfather, Erasmus Darwin, explained the fossil record as a record of evolution. Species descend from other species over long periods of time.
Evolution can also be understood as a process that results in change of the genetic composition of a population over time. A lot of things change through time: a monkey learns to steal food from your house; a tree leans away from the direction of wind; a young man starts lifting weights and builds up his biceps. The examples given are called acquired characters, and they are not evolutionary changes.
Evolutionary changes must be inherited from one generation to the next. Antibiotic resistance in bacteria, and seed-eating birds developing thicker and stronger beaks over generations, are examples of evolution. In both these cases, traits or changes, such as resistance towards an antibiotic or developing a thicker beak, are heritable.
Why do we have to care about evolution?
We mentioned that evolution is central to biology. Understanding evolution is important for your general education. But why do we have to care about it? Does it affect us directly in any way? An understanding of evolution is crucial for scientists who design vaccines for Covid-19 virus; to know how climate change causes species extinctions; to conserve endangered species; to develop new crop varieties, and many other uses.
Vaccine development starts with a knowledge of the virus’s ancestor and how it has changed subsequently. An understanding of the selection pressures on small populations is required to conserve endangered species. To develop new crop varieties, we need to understand which traits are better suited to an environment. We will examine several of these concepts in the following sections.
IV.3 What is natural selection?
Natural selection is one of the processes by which evolution take place. It appears as if nature ‘selects’ organisms that are better suited to survive in a particular environment. But this is not what happens. Darwin made three important claims for natural selection to operate on populations:
- variation: individuals in a population vary in their traits
- heritability: these traits or characters are heritable (they have a genetic basis)
- differential survival and reproduction: individuals with beneficial traits are more successful at surviving and reproducing than others.
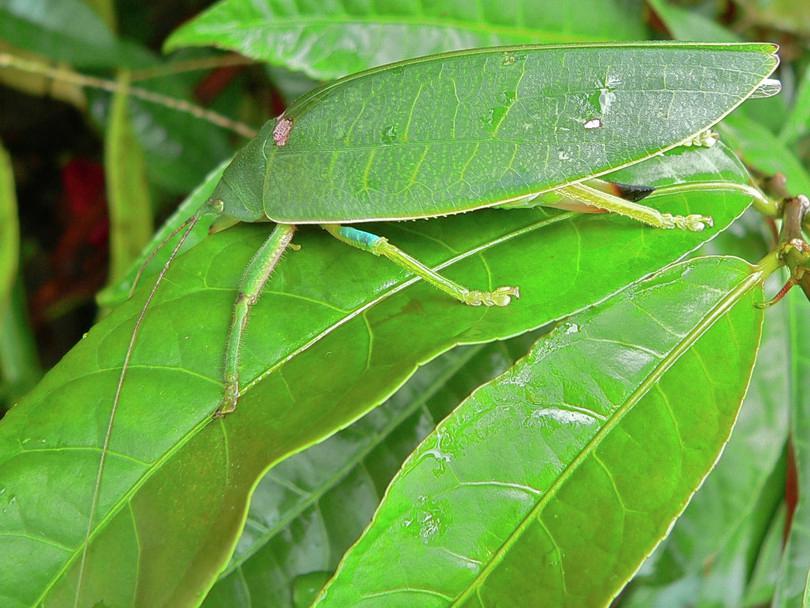
Figure IV.2 Leaf-mimicking insect, a product of natural selection.
Bernard Duponte, Wikimedia commons, CC-BY-SA 2.0.
- adaptations
- Traits (physiological, structural or behavioural) of an organism that allow it to adjust to its environment, which enable better survival and reproduction in that environment. See also: traits.
The leaf-mimicking insect shown in Figure IV.2 is a fantastic example of natural selection. The colour and the patterns on the wings of the insect resembling the venation of a leaf are heritable traits. These traits are adaptations that improve the ability of leaf-mimicking insects to evade predators, survive and reproduce in the environment.
One important point to understand is that although natural selection acts on individuals interacting with their environment, individuals themselves do not evolve. It is the population that evolves over time.1
Consider a population of leaf-mimicking insects in an area. Individuals vary in the extent to which they resemble a green leaf (Figure IV.3). The trait ‘look-like-a-green leaf’ helps insects escape predation. The best-adapted individuals are more likely to survive and reproduce than individuals that look less like a green leaf.
Over many generations, the proportion of individuals with the advantageous trait has increased in the population (Figure IV.3). Genetic variation in the ‘look like a green leaf’ trait makes evolution possible. What this does not mean is that an individual in its lifetime starts looking more and more similar to a green leaf. You can learn more about natural selection by going through these links.
Figure IV.3 Changes in gene frequencies in a population of leaf-mimicking insects.
- allele
- Variation of a gene present at a particular locus on a chromosome, producing variable phenotypes. See also: traits.
In the example of leaf–mimicking insects, we are focusing on evolutionary change within a population. Changes in gene (or allele) frequencies over time results in evolution. Alleles are variant forms of genes. Alleles that produce favourable traits will increase in the population, since individuals that have those alleles are more likely to survive and reproduce.
Natural selection is just one of the processes that causes gene frequencies to change over time. Mutations, genetic drift and migration are other mechanisms. Play this simulated game to understand various factors (environment, predators, resources, genes, population) that influence natural selection.
Directional, stabilising and disruptive selection
Natural selection can change the frequency distribution of heritable traits in three different ways, depending on which phenotype is selected in a population (Figure IV.4).1
Directional selection
If natural selection favours a phenotype that is at one end of the spectrum of existing variation in a trait, then it is directional selection. Directional selection causes an increase in the frequency of the favourable allele in a population.
Disruptive selection
If selection favours phenotypes at both ends of a spectrum, it is called ‘disruptive’. The consequence of disruptive selection is an increase in genetic variation of a population.
Stabilising selection
If selection removes phenotypes at both ends of the spectrum, and preserves intermediate phenotypes, then it is called ‘stabilising selection’. Genetic variation in such populations is reduced. The Rice chapter describes how rice evolved through stabilising selection.
Regardless of the type, natural selection always favours individuals whose heritable traits provide them with higher survival and reproductive success than do traits of other individuals. Looking at the kind of selection that occurs in a population allows us to see how a population might change over time. Stabilizing selection works against evolutionary change since it reduces variation in the population. Directional selection promotes evolutionary change as the population gene pool is comprised of a new allele. Disruptive selection promotes speciation which is also an evolutionary change.
Figure IV.4 Different types of selection. The red and yellow areas denote the current and past frequency distribution of a phenotypic trait among individuals in a population. The arrows indicate where the optimum is and thus the trait characteristics that will be positively selected. All other trait characteristics are under negative selection.
Is natural selection always slow?
You might think the process of natural selection is always slow, taking thousands of years to result in evolution. This is true in some cases, but there are several examples that suggest rapid evolution. A well-known example is the evolution of Galapagos finches. In as little as 30 years, Peter and Rosemary Grant observed evolutionary changes in the finch populations.2 Yet another example is evolution of antibiotic resistance in microbes. Watch this youtube clip to understand how antibiotic resistance can evolve within weeks!
Many factors can influence rapid evolution including short generation time of the organism, large population size, and rapid changes in the environment.
Does natural selection always lead organisms towards perfection or complexity?
It may appear that insects in Figure IV.2 are perfect in terms of their resemblance to leaves. However, this doesn’t mean that all leaf-mimicking species will evolve towards ‘perfection’. If the environmental context changes, a particular trait may no longer be functional or advantageous for an organism. For example, a predator may use smell or movement to detect and eat these leaf-mimicking species. The visual ‘camouflaging’ trait may no longer be advantageous for the insect. So organisms with traits beneficial in one context may be poorly suited to survive in a different context.
Natural selection doesn’t always make organisms more complex. Flightless birds like ostriches have smaller wings than their flying ancestors; horses have fewer toes than their ancestors. At the genomic level, many morphologically ‘simple’ organisms like a maize plant or a water flea might have more complex genomes than humans. These examples tell us that evolution does not always result in increase in complexity.3
Are humans still evolving?
While we have given an example of an insect adapting to its surroundings, what about humans? Is there evidence for human adaptations?
Tibetans’ adaptations to high altitude is one of the finest examples of natural selection in action. Tibetans live at altitudes of 4000 metres and above where oxygen supply is limited (Figure IV.5). Low amounts of oxygen in the bloodstream will cause serious physiological problems to lowlanders, but not for Tibetans!
DNA evidence suggests that Tibetans inherited a few genes from their ancestors who interbred with Denisovans, extinct cousins of Neanderthals around 40 000 years ago.4 These genes are involved in the regulation of haemoglobin production in our body. Tibetans’ physiology includes several biochemical adaptations that allow their bodies to use oxygen extremely efficiently, and thereby need less haemoglobin in their blood. Many thousands of years ago, Tibetans would have varied in their ability to survive at high altitude. Those with Denisovan alleles regulating haemoglobin production were more likely to survive and reproduce than those without Denisovan alleles. The proportion of the population with Denisovan alleles increased and the alleles spread throughout the Tibetan population. This is an example of how modern humans have adapted to new environments by interbreeding with other hominins.
IV.4 What is genetic drift?
- genetic drift
- A mechanism of evolution that occurs by chance, in which some individuals with a specific allele reproduce more than other individuals, despite the survival value of that allele. See also: allele.
We saw that natural selection can change the frequency distribution of alleles in a population. But chance alone can also change the distribution of alleles. This is known as genetic drift. Just by chance, some individuals in a population will survive and have more offspring than others, and this need not be due to an advantageous heritable trait. Larger populations are buffered by the effect of drift, but its effect is pronounced in smaller populations.
Let us say there are only ten individuals in a population, and one of them dies without leaving any offspring. Then none of this individual’s genes will get passed on to the next generation. This is 1/10th of the gene pool of that population – a considerable loss in the genetic variation. However, the effect of one individual not reproducing is much reduced if the initial population was 100 or 1000 to begin with. Drift reduces the genetic variation in a population. Read this article to learn more about genetic drift.
Why are biologists so concerned about genetic drift? Smaller populations face two dangers: over time, they may be highly inbred (mating between related individuals), and they have less genetic variation. Because of this, small populations have a reduced ability to fight diseases or adapt to changing environmental conditions. Think about how this may affect species that are already endangered. Read about why conservation biologists are worried about the African cheetahs.
IV.5 What is artificial selection? How is it different from natural selection?
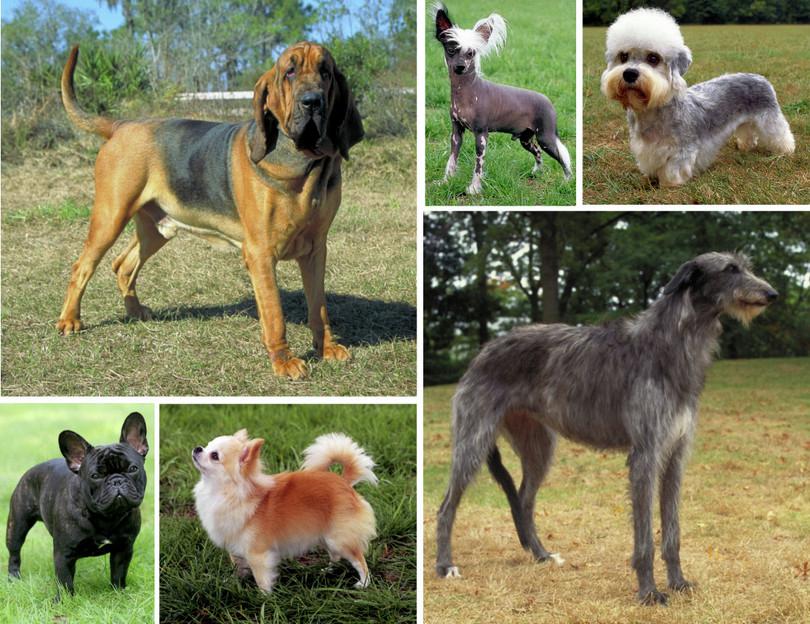
Figure IV.6 Dog breeds, a product of artificial selection.
Mary Bloom, Wikimedia commons, CC-BY-SA 4.0.
- artificial selection
- The process undertaken by humans to identify desirable traits in organisms and arrange the reproduction of those traits in future generations. See also: traits.
Artificial selection is a process by which humans selectively breed organisms with certain desirable traits. It is the same as natural selection, except that humans, rather than environmental pressures, select which individuals to breed. Farmers and breeders have been doing this for thousands of years.
Various dog breeds present today are a prime example of artificial selection (Figure IV.6). Dogs are descendants of the gray wolf, but there is little resemblance between the two. Humans have selected for different behavioral traits (tameness, endurance, and so on), and morphological traits (coat colour and texture, height, and so on).5 Similarly, the tomatoes we have today are products of artificial selection. What kinds of qualities or traits would you want in a tomato? Large, juicy and pulpy are some preferred traits.
The Rice chapter refers to wild rice that grows only in floodplains, while the domesticated rice varieties have been artificially bred to grow in various environments. The Cotton chapter illustrates how humans have selected traits in cotton varieties to increase yield and fibre quality. Artificial selection is powerful: you can pick and choose the traits you want in organisms and remove the traits you do not want.
IV.6 How are new species formed?
A species (singular and plural are the same) is a group or population of individuals that look alike and can interbreed in nature. Classifying organisms as a species is not always straightforward because some species go through different stages in their life cycle (think of caterpillars, pupae and adult butterflies). The concept of a species is something that humans developed for our own convenience. You can study various ways of defining a species in the Western Ghats chapter.
- reproductive isolation
- The process by which biological properties found in organisms prevent populations from interbreeding. Mechanisms of reproductive isolation include ecological isolation and temporal isolation, among others.
How do new species evolve? Remember, new species can arise if individuals from one population do not mate and reproduce with individuals from another population of the same species. This is called reproductive isolation.
- gene flow
- The transfer of genetic material or alleles from one population to another of the same species, thereby altering the allele frequency or genetic variability of the receiving population.
Movement of individuals (gene flow) between populations prevents the formation of new species. Factors that reduce gene flow could be external (geographical barriers such as mountain ranges, rivers, gorges and continental drift) or internal (differences in mating times, mating location, or lack of ‘fit’ between sexual organs). Reduced gene flow facilitates speciation. Read more about how new species are formed in watching this video on Anole lizards.
- allopatric
- Existing or occurring in isolation or geographic separation. See also: sympatric.
Speciation that happens due to geographical isolation is called allopatric (allo=other, patric=fatherland) speciation. That is, if a single species becomes geographically separated, each population can eventually give rise to new species.
- sympatric
- Existing or occurring in the same geographical area. See also: allopatric.
- niche
- The abiotic and biotic conditions necessary for an organism to persist, and the organism’s role within the ecosystem.
Speciation that occurs within the range of an ancestral population is called sympatric (sym=same, patric=fatherland) speciation. This may seem difficult to imagine at first. How can one reduce mating and reproduction when living in the same area? Exploiting new niches to live or having abnormal numbers of chromosomes so that the offspring are no longer viable are some ways that organisms achieve sympatric speciation.
The black-necked stork, Painted stork and Asian Openbill are three sympatric stork species found in Keoladeo National Park in Bharatpur, Rajasthan (Figure IV.7). These species forage at different water depths, and feed on different prey, thereby reducing niche overlap.6 They do not interbreed, therefore they are separate species. Although these three stork species are categorized as sympatric species, we do not know the time of speciation. They should be viewed as an example of similar species that show niche separation.
IV.7 What are phylogenetic trees and how do we read them?
A phylogenetic tree can be thought of as a ‘family tree’ that represents evolutionary relationships among organisms (Figure IV.8). Scientists use phylogenetic trees to: a) classify organisms, and b) understand the relationship between extinct, extant (living) and ancestral species. A phylogenetic tree is a hypothesis (a most probable explanation) about how species are related to each other. It is a hypothesis because we cannot be 100% sure about what has happened in the past.
Evidence for building a tree comes from studying characters or traits of species, by using morphological and molecular information. So, your tree is as good as the data you have. In the Rice chapter, you will be introduced to dendrograms. They are also phylogenetic trees, and biologists use these terms interchangeably. Let us understand a little bit more about phylogenetic trees, and some basic rules to build them.
- clade
- A group of organisms that encompasses all the descendants that have evolved from a common ancestor.
With the help of a primate phylogenetic tree (Figure IV.8), we have outlined some basic terminology that you need to know. The numbered boxes in Figure IV.8 are described below:
- Outgroup: An outgroup is a lineage that falls outside the clade being studied, but closely related to that clade.
- Root: A root is the branching point that represents the last common ancestor of all the other lineages on the tree.
- Ingroup: An ingroup is a clade that is the focus of a phylogenetic analysis. A clade is a group that includes a single ancestor and all of its descendants.
- Node: A node is a branching point on a tree. Each node represents a speciation event.
- Character: A character state change denotes a lineage in which a new character state has evolved.
- Terminal taxon: A terminal taxon appears as a branch tip in a phylogenetic tree. It may be an extinct or extant species.
- Sister group: Sister groups are two clades that are each other’s closest relatives.
Evolutionary time is read from the root of the tree to the tips (most recent times).
- homologous
- In reference to body parts or organs that share evolutionary origins but carry out different functions. See also: analogous.
- analogous
- In reference to body parts or organs that have developed as independent adaptations to carry out similar functions, but do not share evolutionary origins. See also: homologous.
In order to build trees, you need to select characters that are homologous (derived from a common ancestor) rather than characters that are analogous (derived independently) to each other. Characters should be shared by some, but not all groups being studied. And all characters should be evolving independently. You can learn more about tree building by playing this game.
The phylogenetic tree in Figure IV.8 tells us about our own relationship with other primates. Understanding primates that are alive today will help us to understand how we evolved. Scientists group primates into Prosimian or first apes that are largely nocturnal, with a keen sense of smell, and Anthropoid, ‘man–like’ apes that are largely diurnal (active in the daytime) and are largely visual. Anthropoid primates are further grouped as monkeys (with tails) and apes (without tails). Humans have many traits that are unique, and are different from those of our closest cousins. Evolution accounts for both unity and diversity of life, and the match between organisms and their environments.
-
Reece, JB, Urry, LA, Cain, ML, Wasserman, SA, Minorsky, PV and Jackson, RB, Campbell Biology 10th ed. (Boston: Pearson Education, 2014). ↩ ↩2 ↩3
-
Grant BR and Grant PR, Evolutionary Dynamics of a Natural Population: The Large Cactus Finch of the Galapagos (University of Chicago Press, 1989) ↩
-
Martin, JS, Smith, NA and Francis, CD, ‘Removing the Entropy from the Definition of Entropy: Clarifying the Relationship between Evolution, Entropy, and the Second Law of Thermodynamics’, Evolution: Education and Outreach 6, no. 1 (2013): 1–9, doi: 10.1186/1936-6434-6-30. ↩
-
Simonson, TS et al., ‘Genetic Evidence for High-Altitude Adaptation in Tibet’, Science 329(5987) (2010): 72–75, doi: 10.1126/science.1189406. ↩
-
Adams, JU, ‘Genetics of Dog Breeding’, Nature Education 1, no. 1 (2008): 144, accessed 23 September 2021. ↩
-
Ishtiaq, F, Javed, S, Coulter, MC and Rahmani, AR, ‘Resource Partitioning in Three Sympatric Species of Storks in Keoladeo National Park, India’, Waterbirds 33, no. 1 (2010): 41–49, doi: 10.1675/063.033.0105. ↩